Silicon Carbide Power Modules Maximize Active Front End Efficiency
Article
Engineers designing UPS with great care to ensure smooth enterprise data center operation 24/7 are also aware that their power supplies are destined to be part of a setup that gulps 90 TWh of U.S. electricity every year — enough to sustain 30 large and noxious coal-fired plants. Power engineers in another design camp, working to ensure their fast chargers can speedily top up EVs, are also aware of the cost of electricity and the environmental impact of its generation.
Engineers targeting any application area are joined in their concerns over efficiency, power density, and cost. And, even if they have yet to design with it, they are aware that the solution may lie in Silicon Carbide (SiC) technology.
This paper addresses these concerns and, by doing a side-by-side comparison, demonstrates that Silicon Carbide (SiC) is by far the better choice over silicon (Si)-based devices for high power applications. The demonstration uses an essential part of UPS and charger systems, the active front end (AFE), to explore improvements in size and power density, power losses and efficiency, and bill of materials (BOM) costs.
The paper thus aims to turn that general awareness of SiC benefits into a clearer understanding, clearing a path through an entrenched less-efficient technology toward greater SiC-based design experience.
The broad challenge
The challenge in AFE design can be broadly expressed as a wish list of changes an engineer would want:
- Lower switching and conduction losses in the semiconductor devices
- Smaller and lighter cooling system
- Smaller and lighter passives — capacitors and inductors
- All of the above with reduction in operational cost as well as BOM cost
Any technology that resolves all of these challenges — simultaneously — can indeed have a significant impact on product competitiveness as well as the environment.
Why Silicon Carbide?
Silicon Carbide enables engineers to check items on the list above by virtue of material and resulting device properties.
Compared with the traditional Si technology, SiC devices offer a 2-3X lower on-state voltage drop than Si, thus lowering conduction losses in SiC switches. Since SiC devices are majority carriers, they offer much higher edge rates (di/dt) than possible with Si. Their 10X higher breakdown field than Si allows SiC devices to withstand higher voltage in the same package.
A higher thermal conductivity of 3.3-4.5 W/cmK versus Si’s 1.5 W/cmK enables SiC devices to conduct heat away much more quickly, helping to reduce cooling requirements in the system. Moreover, SiC chip temperatures can reach 250-300°C (versus Si’s 125°C) and junction temperatures in Wolfspeed devices can go up to 175°C before affecting reliability. This means that the devices can run hotter and with a smaller cooling rig.
Wolfspeed’s SiC power modules offer the following advantages over Si versions:
- They are application-targeted with module selections offered in a variety of voltage and current ratings, and form factors, as well as with switching and conduction optimization
- They have lower RDS(ON) compared with IGBT modules
- They offer faster switching speeds
- They have lower switching losses
Application advantages of the AFE topology
The AFE is applicable to almost all grid-tied converters. Two prominent topologies in today's emerging markets are shown in Figure 1. The double-conversion UPS architecture comprises an AFE or rectifier, a DC/DC converter and an inverter. In normal power flow, a small current goes into the DC/DC converter that maintains the battery charge. Most of the power is sent through the DC link into the inverter where it feeds the load.
Under a power fault, the AFE stops switching and the DC/DC converter sends power from the battery into the inverter to feed the load. Some applications may use the battery also to compensate for poor load or grid-side power quality.

In the off-board DC fast charger as well, the AFE connects the converter to the grid. It rectifies the grid voltage into a stable DC link voltage, which then can be used to charge the batteries. The off-board charger topology is simpler with the AFE interfacing directly with just the DC-DC converter to quickly charge the EV.
In both applications, the AFE uses three half-bridge power modules – one for each phase.
Defining the problem & design goals
A key issue with IGBT-based AFEs is that they are large and inefficient. They have high switching losses and, because they are also significant heat sources, engineers have the option to either use bulky cooling systems or take a performance hit in order to lower the heat generated. But, although demands vary slightly, all customers want to pay for a high efficiency system, not a heater.
The AFE design goals, therefore, can be defined as:
- Regulate the DC link voltage under normal operation by controlling the input current magnitude
- Minimize power quality issues by sourcing very low THD (<5%) current with very high-power factor
- Minimize BOM component costs
- Shrink system volume to enable more compact systems
- Maximize efficiency
With this in mind, IGBT and SiC variants of an AFE system were designed to output 200 kW of high-quality rectified power with a well-regulated DC bus.
IGBT- vs SiC-based designs
The IGBT- and SiC-based systems are presented broadly before delving deeper with a side-by-side comparison of the component sizes and losses.
Si-based high-power designs, like the AFE example, typically use IGBTs. Shown in Figure 2 is the circuit diagram with the power module and its physical cooling requirements. To use a best-in-class component, a module was chosen from among the dominant IGBT modules today that come in EconoDUAL® package. The topology requires three such power modules — each red box shown in the figure includes a single power module, a heatsink, and two fans.

The system could be optimized to switch at a frequency as high as 8 kHz while needing a 100 µH inductor. For a 40 °C ambient temperature, the IGBT junction temperature (Tj) reaches 130 C and the separate diode chip junction temperature reaching 140 °C. This required a large heat sink and two fans per module even after limiting the switching frequency to 8 kHz.
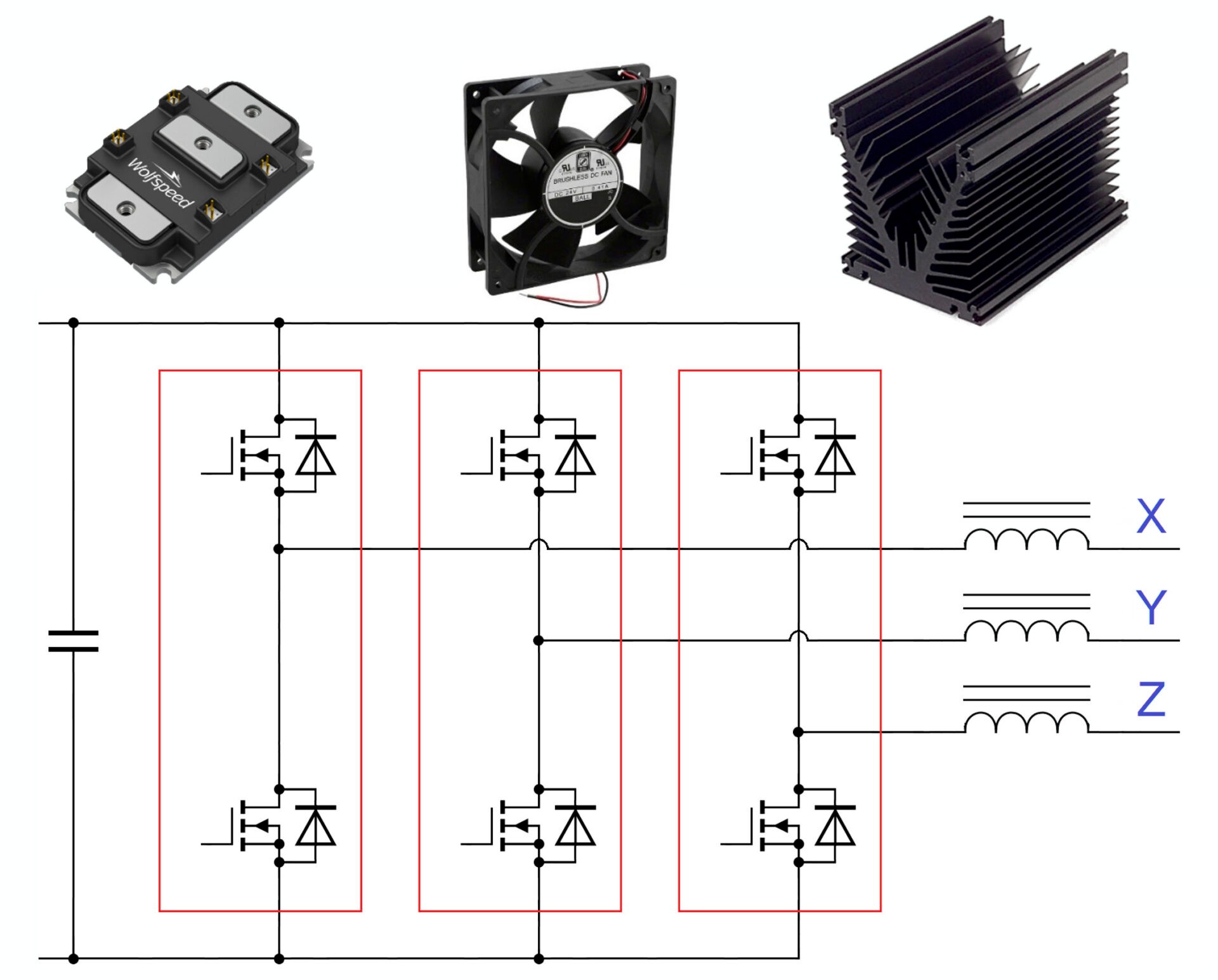
The SiC-based system used a Wolfspeed XM3 power module, the XAB400M12XM3. The system can switch at a much higher 25 kHz, and uses a 30 µH inductor. For the same 40 °C ambient temperature, the MOSFET junction temperature reaches 164 °C. Again, each red box shown in Figure 3 comprises the module and it’s much lower cooling requirements.
Power modules compared
Wolfspeed’s XM3 power module platform occupies 60% less volume and 55% less area than an equivalently rated 62-mm module. Compared with a similarly rated EconoDUAL® IGBT module, the reduction in size, volume, as well as weight is significantly more.

The XM3 platform’s key features include:
- A high power density of up to 32 kW/L
- Junction temperature of up to 175°C
- Low inductance (6.7 nH)
- >5X lower switching losses
- Low conduction losses without intrinsic knee-voltage
- High reliability silicon nitride power substrate for enhanced power cycling capability
In the AFE under consideration, Table 1 compares the IGBT power module losses against the CAB400M12XM3.
Type of Loss | IGBT Module (W) | XM3 Module (W) | |
---|---|---|---|
IGBT/MOSFET | Conduction | 16 | 132 |
IGBT/MOSFET | Turn-on switching | 66 | 96 |
IGBT/MOSFET | Turn-off switching | 211 | 91 |
IGBT/MOSFET | Reverse recovery | N/A | 12 |
IGBT/MOSFET | IGBT/MOSFET total | 293 | 331 |
Diode | Forward conduction | 140 | N/A |
Diode | Reverse recovery | 122 | N/A |
Diode | Diode total | 262 | N/A |
Module Total | 555 x 2 = 1110 | 331 x 2 = 662 |
Table 1: The loss comparison demonstrates a per module loss reduction by SiC of 40% over IGBT.
As shown in Table 1, using Wolfpseed SiC technology helps overcome the first broad design challenge by reducing the total switching and conduction losses, which enables the remaining broad challenges to be addressed. Note that the Wolfspeed SiC MOSFET’s inherent body diode has a reverse recovery charge (Qrr) that is less than 1% of that of Si’s. In an attempt to somewhat alleviate this problem, IGBT modules include a separate diode that separately and additionally contributes to losses.

Smaller, lighter cooling system
The high MOSFET junction temperature allowed by Wolfspeed’s SiC technology and the XM3’s low losses have an immediate effect on the cooling requirements.
With a loss per module of 1.11 kW, every EconoDUAL® needs to be mounted on a large heatsink with a pusher and puller fan each to achieve sufficient airflow for cooling efficiency. The cooling system volume is 6.4 L/module.
Given the 40% lower losses, the XM3 needs a smaller heatsink and just one fan to achieve the same result (@40 °C). The cooling system volume is just 3.7 L.
This 42% reduction in cooling system volume is accompanied by yet another advantage — a 70% reduction in the AFE system thermal solution cost.
The impact on passives
By enabling an increase in the switching frequency by a factor of three, from 8 kHz to 25 kHz, the SiC-based AFE needs smaller passives (Figure 6).
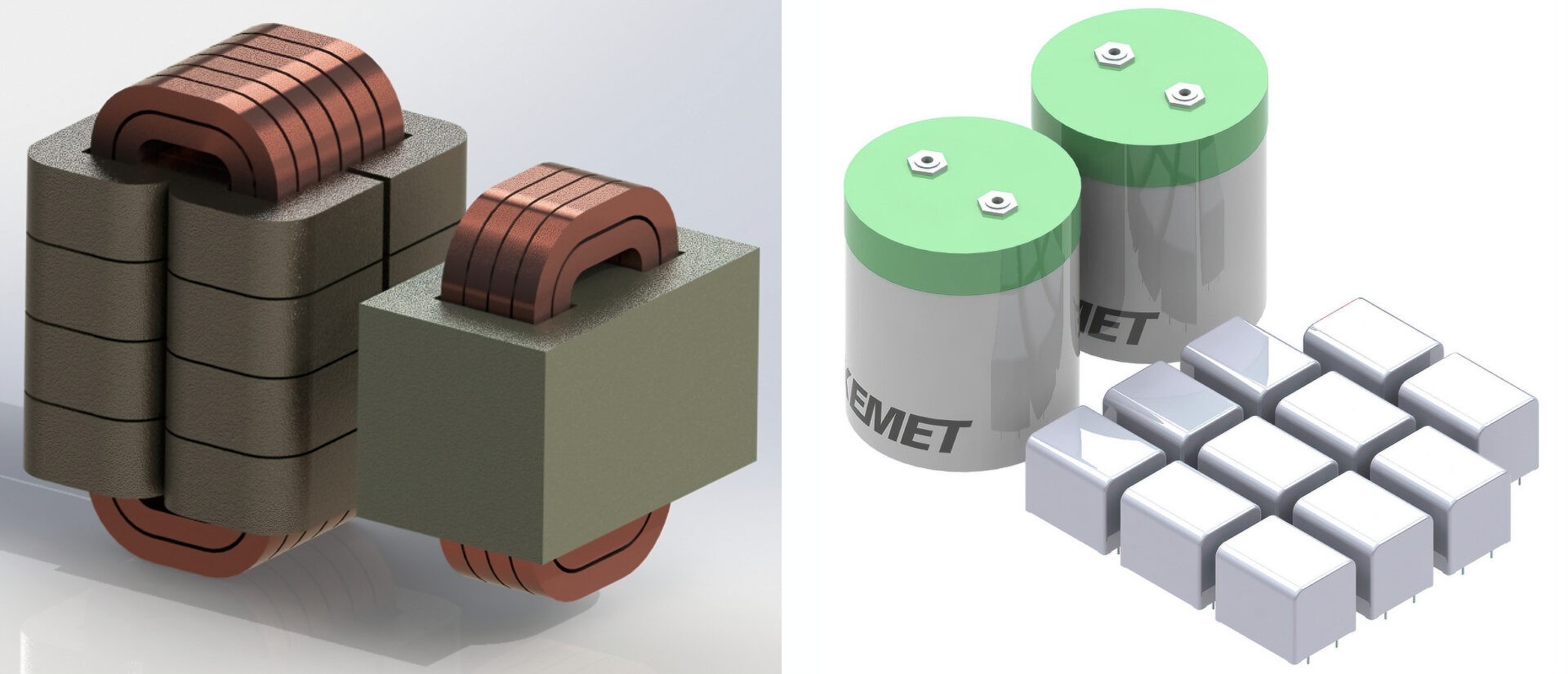
As mentioned earlier, the required inductance can also be reduced by a factor of three, from the IGBT design’s 100 µH to 30 µH. The resulting reduction in the physical size is about 37%. Moreover, I2R losses in the inductor are also reduced by close to 20%.
For the power levels required by the AFE example, the cost of the magnetics, including the core and the copper windings, is lower in the XM3 design by 75% over the IGBT-based AFE.
The effect on the required DC link capacitance is similar due to the increased switching frequency. While for the IGBT variant 1800 µF are required, the SiC MOSFET-based design only needs 550 µF capacitance. The side-by-side comparison in Figure 6 illustrates the reduction in the volume of the needed capacitance by 54%.
AFE system-level comparison
At the system level, the 3X increase in switching enabled by SiC translates to a 3X improvement in control bandwidth that in turn means faster response time to dynamic conditions. The easing up of the demand on passives, including the cooling system, results in a 37% reduction in the BOM costs toward those components put together.
The SiC-based AFE also has 40% lower losses than the IGBT-based system. For a system that runs continuously — 24 hours a day, 7 days a week — this leads to 26 MWh in annual energy savings. Beyond the green credentials, at a cost of $0.10/kWh, SiC can lower the annual operational cost by $2,591.
Looking beyond performance, passive BOM costs, and operational costs, the SiC-based system is much smaller in size and weight. It effects a system volume reduction of 42% over the IGBT version (Figure 7).

Conclusion
A side-by-side comparison of best-in-class IGBT EconoDUAL® and Wolfspeed CAB400M12XM3 SiC-MOSFET power modules in similarly rated AFE systems reveals that SiC technology makes the aforementioned designer’s wish list come true. Wolfspeed’s XM3 platform helps significantly increase efficiency throughout the system, boost overall system response and performance, cut the system-wide volume to achieve much higher power density, and increase competitiveness by shaving off overall passive BOM costs.
Contact Wolfspeed to learn more about the technology and platform of choice for AFE and other high-power designs.